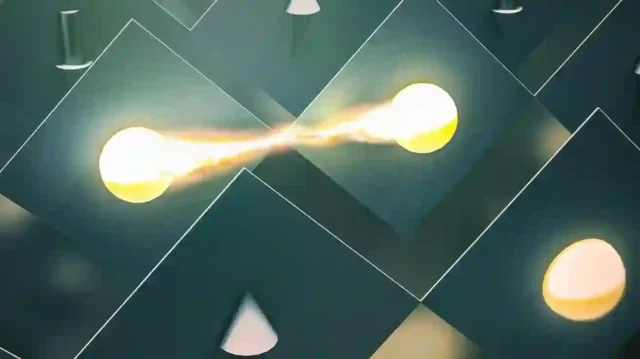
A recent breakthrough by a team of physicists from Stanford University and the SLAC National Accelerator Laboratory has called into question one of the most influential theories in quantum materials science. Their study, published in Physical Review Letters, challenges the validity of the Hubbard model, a theoretical framework that has guided research on electron interactions for over five decades—particularly in the context of high-temperature superconductors known as cuprates.
The team’s findings suggest that the Hubbard model, long considered a promising candidate for explaining how cuprates become superconducting at higher temperatures than traditional materials, fails to account for a surprisingly strong attractive force between electrons in these materials—even in simplified, one-dimensional forms.
High-Temperature Superconductors: A Persistent Puzzle
Superconductivity—the ability of a material to conduct electricity without resistance—was first discovered in the early 20th century in elements like mercury at extremely low temperatures. While these materials are fascinating, their requirement for near absolute-zero conditions makes them impractical for widespread use.
Enter cuprates: a family of copper-oxide compounds that shocked the scientific world in the 1980s by demonstrating superconductivity at temperatures far above the norm, around -138°C. Though still far below room temperature, this leap opened the door to real-world applications using cheaper cooling agents like liquid nitrogen. However, while the phenomenon has been observed, the exact mechanism behind high-temperature superconductivity in these materials remains unsolved.
Cracks in the Hubbard Model
For decades, the Hubbard model has been the go-to theory to describe how electrons interact in materials like cuprates. The model captures the competition between electrons’ tendencies to move freely and their tendency to repel each other when occupying the same space. In simple terms, it tries to explain how electrons behave when they are both social and territorial.
Yet despite its wide use, the Hubbard model has never been conclusively proven to explain high-temperature superconductivity in cuprates. The mathematical complexity of the model, coupled with the intricate nature of cuprate materials, has made it difficult to validate through experimentation—until now.
In a previous 2021 study, SLAC researchers took a novel approach by focusing on a one-dimensional version of a cuprate system. By reducing the problem to a single chain of atoms, the team was able to strip away some of the computational and conceptual complexity. Using high-intensity X-rays, they examined quasiparticles known as holons—entities that represent the charge aspect of an electron—and discovered that electrons were attracting each other with a force ten times stronger than predicted by the Hubbard model.
Adding a New Piece to the Puzzle: Spinons
Building on that earlier work, the team expanded their research to study spinons—quasiparticles that reflect the spin characteristics of electrons. This time, they aimed to see if the unexpected force observed in the holon study would also appear in spin behavior.
To investigate, they created a synthetic one-dimensional chain of doped cuprate atoms using facilities at the Stanford Synchrotron Radiation Lightsource, and then conducted X-ray scattering experiments at advanced synchrotron facilities in the U.K. and New York.
Once again, the team found that electron interactions could not be fully explained by the Hubbard model. But when they included an additional attractive force in their calculations—like the one discovered earlier—the data from the spinon study lined up far more closely with experimental observations.
“This is strong evidence that the Hubbard model, even in its simplest 1D application, does not capture the full physics of cuprates,” said Dr. Wei-Sheng Lee, a SLAC scientist involved in the project. “If it can’t describe what’s going on in one dimension, there’s little hope it will succeed in the more complex two-dimensional scenarios where actual high-temperature superconductivity takes place.”
Searching for the Missing Ingredient
With two independent experiments now pointing toward an additional, previously unaccounted-for attractive force, the scientific community is left with an intriguing question: What is the origin of this force?
Dr. Thomas Devereaux, a senior physicist at SLAC and Stanford who oversaw the theoretical aspects of the research, believes the answer may lie in phonons—the vibrations of the atomic lattice that holds the material together. In traditional superconductors, phonons are known to play a key role in helping electrons form the pairs necessary for zero-resistance flow. If a similar mechanism is at play in cuprates, it would point to a long-overlooked bridge between classic and high-temperature superconductivity.
However, Devereaux and his team emphasize that much more research is needed to confirm this hypothesis. Future experiments will likely explore the relationship between these electron-vibration interactions and the conditions under which superconductivity arises.
Implications for Future Technologies
The implications of this research could be vast. Unlocking the secrets of high-temperature superconductivity would be a game-changer for technologies ranging from magnetic levitation trains to lossless power grids and even scalable quantum computers.
By revealing the limitations of the Hubbard model, this study challenges theorists to reexamine their assumptions and pushes the field toward a deeper understanding of electron dynamics in complex materials. “We’re building on the work of generations of scientists,” said Jiarui Li, the study’s lead author and a postdoctoral researcher at SLAC. “There’s still a long way to go, but every step brings us closer to practical superconductors and a new era of energy and computing.”
Looking Ahead
As researchers continue probing the quantum behavior of cuprates, the focus now shifts to identifying new theoretical frameworks that can better account for these anomalies. Whether the answer lies in phonons or some yet-unknown interaction, one thing is clear: a 50-year-old theory has reached its limit.
And with that revelation, a new chapter in superconductivity research is just beginning.
—
This study, titled “Doping Dependence of 2-Spinon Excitations in the Doped 1D Cuprate Ba2CuO3+δ,” was conducted with support from the U.S. Department of Energy and utilized facilities at SLAC, Brookhaven National Laboratory, and the Diamond Light Source in the UK.